Day 1 :
Keynote Forum
Francesca Storici
Georgia Institute of Technology, USA
Keynote: Mechanisms of RNA-guided DNA repair and modification
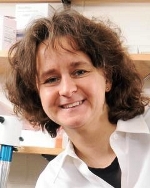
Biography:
Francesca Storici is Professor in the School of Biological Sciences at Georgia Tech. She received her Ph.D. in Molecular Genetics from the International School of Advanced Studies in Trieste, Italy (1998). She was a postdoctoral fellow at the National Institute of Environmental and Health Sciences (NIEHS, NIH) (NC) till 2007, and then research assistant professor at the Gene Therapy Center of the University of North Carolina at Chapel Hill, NC. She joined the Georgia Institute of Technology as an assistant professor in 2007, and became Distinguished Cancer Scientist of the Georgia Research Alliance. In 2013 she was promoted to Associate Professor with tenure. In 2016, she became Howard Hughes Medical Institute Faculty Scholar. In 2018, she was promoted to Professor. Her research is on DNA damage, repair and gene editing.
Abstract:
We recently discovered that RNA serves as template to repair DNA double-strand breaks (DSBs), either indirectly, in the form of complementary DNA (cDNA), or directly, in the form of transcript-RNA in budding yeast. By removing the function of ribonucleases (RNases) H1 and H2, which cleave RNA in RNA-DNA hybrids, we found that transfer of genetic information from RNA to DNA occurs with an endogenous generic transcript in cis, and is thus a more common mechanism than previously anticipated.
DSB repair by RNA requires the recombination protein Rad52 but not Rad51, or Rad59. Upon overexpression of yRad52, yeast or human Rad52 N-terminal domain (NTD), the frequency of DSB repair by RNA increased up to of a factor of 68. These results indicate that human Rad52 could potentially catalyze DSB repair by RNA also in human cells. Moreover, in the absence of SAE2 or EXO1 genes, which are important for DNA end resection, the frequency of DSB repair by RNA was either increased or not changed, respectively. DSB repair by RNA occurs in the absence of a bona fide reverse transcriptase (RT) enzyme. Nevertheless, overexpression of RT stimulates DSB repair by RNA. These results support an RNA-dependent mechanism of DSB repair mediated by Rad52 that catalyzes a reaction in which RNA invades a broken double stranded DNA in conditions of limited end resection (Figure 1). While, the recombination protein Rad52 is a key factor for RNA-templated DNA repair of DSBs, RNA can modify DNA even without the induction of a DSB in a Rad52-independent manner. Our results suggest that transcript RNA, like non-coding RNA, may have a significant role in genome stability and genome modification, much more prominent than previously anticipated.
We acknowledge support from NIH GM115927, NSF-MCB-1615335, and HHMI grant 55108574 to F.S.
Keynote Forum
Razvan Chereji
NICHD, NIH, USA
Keynote: Precise genome-wide mapping of single nucleosomes and linkers in vivo
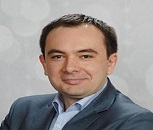
Biography:
Razvan Chereji holds a Ph.D. degree in physics from Rutgers University and currently he is a research fellow at the National Institute of Child Health and Human Development, NIH. He studies physical models that describe the chromatin organization, its dynamics, and the interactions between DNA and DNA-binding proteins, including histones, transcription factors, polymerases and chromatin remodelers.
Abstract:
In eukaryotes, nucleosomes are the basic units of DNA packaging. Micrococcal nuclease (MNase) digestion followed by sequencing (MNase-seq) is the most used method of mapping nucleosomes. Unfortunately, MNase digests different sequences with different rates, and nucleosome maps are affected by the degree of digestion. Moreover, other proteins also offer protection against MNase and can be mistaken for nucleosomes in MNase-seq experiments.
To eliminate the biases introduced by MNase, we have developed a chemical cleavage method that allows us to precisely map both single nucleosomes and linkers with very high accuracy genome-wide in budding yeast. We confirm that S. cerevisiae promoters are sites of strong nucleosome depletion but attribute the putative nucleosome depletion seen at termination sites to MNase bias. Our nucleosome mapping data has the highest resolution among the currently available techniques, and this accuracy allows us to distinguish alternative rotational positions that nucleosomes occupy in different cells. Furthermore, we show that linker DNA has quantized lengths for individual genes. By comparing our nucleosome dyad positioning maps to existing genomic and transcriptomic data, we evaluate the contributions of sequence, transcription, histone H1 and the H2A.Z variant in defining the chromatin landscape. We show that DNA sequence has a very limited effect on establishing the nucleosome organization as observed in vivo. Moreover, we find that the degree of gene compaction, measured by the spacing between neighboring nucleosomes, correlates with the transcription level, amount of histone H1 bound to the gene, and the amount of H2A.Z variant that is incorporated in the nucleosomes closest to the gene promoter (“+1 nucleosomes”). Furthermore, we present a biophysical model based on simple physical principles, which shows that steric exclusion between neighboring nucleosomes suffices to explain the salient features of nucleosome positioning and the complex nucleosome phasing pattern that is observed near the gene ends.
Keynote Forum
F. Brad Johnson
University of Pennsylvania, USA
Keynote: WNT signaling and telomere capping can be mutually supportive: Potential therapeutic implications
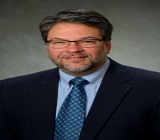
Biography:
Brad Johnson is a Professor of Pathology and Laboratory Medicine at the Perelman School of Medicine at the University of Pennsylvania, where he serves as Associate Director or the Penn Institute on Aging and Assistant Director of the Clinical Immunology Laboratory at the Hospital of the University of Pennsylvania. His research has focused for over twenty years on the basic mechanisms by which telomeres are maintained, and how telomere dysfunction impacts cell and tissue health.
Abstract:
Perturbation of telomere structure, including critical shortening, can cause telomere “uncapping”, leading in turn to disruption of cell and tissue function. Lengthening by the enzyme telomerase is the primary mechanism by which the telomere shortening caused by genome replication and DNA damage is reversed. The consequences of telomere uncapping can be seen in human genetic diseases caused by partial loss of telomerase activity. An example is dyskeratosis congenita (DC), characterized by defects in tissues that depend most on telomerase, including those that naturally turnover rapidly (e.g. hematopoietic cells, GI epithelium) or divide following damage (e.g. lung and liver epithelium). Despite the need to maintain telomere length, even in normal humans telomerase levels are limited and thus telomeres shorten with age in most tissues, a strategy that has evolved evidently because it limits cancer progression.
Recently we uncovered a novel positive feedback loop connecting telomere capping and the WNT signaling pathway in the intestinal stem cell niche. This pathway is used by cells to communicate with neighboring cells, particularly niche cells and the stem cells that they support. We leveraged this loop to rescue intestinal pathology via pharmacologic WNT stimulation in mice lacking telomerase (late generation Terc-/-) and in cultured human intestinal organoids derived from DC iPS cells. Remarkably, rescue involved improved telomere capping, demonstrating that capping can be influenced by extracellular factors and that it can involve length-independent mechanisms. However, WNT is also well established to upregulate expression of the catalytic and limiting component of telomerase, TERT, and thus WNT agonism can also enhance capping via telomere lengthening. Therefore WNT agonism might improve telomere capping by at least two mechanisms in diseases like DC.
The evidence connecting telomeres and WNT signaling will be discussed, along with underlying mechanisms and recent efforts to use WNT and other pharmacologic approaches to rescue to the pulmonary epithelium of cultured DC human lung organoids. Finally, the possibility will be discussed that whereas enhancement of telomerase activity in younger individuals carries a cancer risk, such enhancement in individuals with DC, and perhaps in normally aged people, might have a net anti-cancer benefit.
Keynote Forum
Dipankar Sen
Simon Fraser University, Canada
Keynote: Fishing for G-quadruplexes and G-quadruplex proximal proteins by virtue of the peroxidase properties
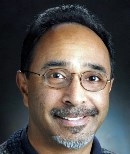
Biography:
Dr. Dipankar Sen was born in 1957 in Calcutta, India, and graduated with a B.A. (Hons.) from Cambridge University. He completed his Ph.D. in Chemistry at Yale University, with Donald M. Crothers and then did postdoctoral work with Walter Gilbert at Harvard University. He has been on the faculty at Simon Fraser University since 1991 and is currently Professor of Chemistry and Professor of Molecular Biology and Biochemistry. His research interests focus on the fundamental chemical and physical properties of DNA and RNA, nucleic acid catalysis, and G-quadruplexes.
Abstract:
The powerful in vitro affinity observed between the ubiquitous cellular cofactor, hemin, and DNA or RNA G-quadruplexes, raises the intriguing possibility that this interaction also occurs in vivo. A further, intriguing possibility is that this affinity, and the notable peroxidase activity of the resulting G-quadruplex•hemin complexes, could be used to tag G-quadruplexes (and G-quadruplex-interacting and -proximal proteins) within living cells. Such investigations are in principle enabled by our long-time observation that hemin•G-quadruplex complexes are strongly enabled towards catalysis of 1- and 2-electron oxidative reactions, with phenolic compounds being especially outstanding substrates. We have recently reported that the use of biotinyl tyramide as a substrate for G-quadruplex•hemin peroxidases in vitro leads to the self-biotinylation of the G-quadruplexes themselves. Such self-biotinylation occurs with good efficiency and high discrimination, being entirely specific for G-quadruplexes and not, for instance, DNA duplexes. Furthermore, DNA biotinylated in this way remains amenable to polymerase chain reaction amplification, rendering it suitable for analysis by ChIP-Seq and related methodologies. We will present new data on this self-biotinylation methodology, that we anticipate will serve as a sensitive tool, orthogonal to existing ones, for identifying, labeling and pulling down cellular RNA and DNA G-quadruplexes as well as proteins either bound to or proximal to such quadruplexes.
- Mechanisms and Regulation of DNA Repair and Replication Proteins
Location: Canada
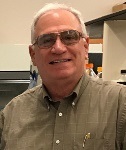
Chair
Michael Leffak
Wright State University, USA
Session Introduction
Marc Wold
University of Iowa, USA
Title: DNA repair depends on specialized DNA-binding by replication protein A
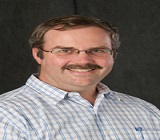
Biography:
Marc Wold My laboratory has been study cellular DNA metabolism for more than 20 years. My lab has experience with a wide-range of biochemical, molecular and cellular approaches for analyzing DNA metabolism in vitro and in vivo. We have extensive experience with purification and biochemical analysis of proteins involved in DNA replication and repair. We have either developed or applied many methods for examining DNA binding and functional analysis of RPA in DNA replication and repair. We also carried out structural analysis of RPA and in cell methods for analyzing the function of different forms of RPA or other proteins in human cells. These studies have developed methodology for determining the functions of RPA in cellular DNA replication, DNA repair (including methods for analyzing the efficiency of specific DNA repair pathways) and checkpoint activation. My lab has generated and purified a large number of mutant forms of RPA and analyzed the mechanism of RPA-DNA interactions. We have domain deletion and point mutations at disrupted the function of individual domains of RPA, alter DNA
Abstract:
Replication protein A (RPA), the major single-stranded DNA-binding protein in eukaryotic cells, is required for processing of single-stranded DNA (ssDNA) intermediates found in replication, repair, and recombination. RPA is a highly flexible complex composed of three subunits, RPA1, RPA2 and RPA3 that contain six DNA binding domains (DBDs). One of the recurring mysteries of RPA is that the affinity of RPA for ssDNA does not directly correlate with cellular function. There are RPA mutations that reduce the affinity of the complex by two orders of magnitude are fully active in vitro and in cells, while other mutations that display higher affinity for ssDNA are partially or completely inactive. In particular, we have characterized a set of separation-of-function mutants in RPA1 that have mutations in pairs of aromatic residues in the DNA binding sites of DBD-A or -B. These mutants support DNA replication but are defective in multiple DNA repair pathways including NER and double strand break repair. These results suggest that replication and repair require different RPA-DNA interactions
We utilized single molecule total internal fluorescence microscopy (smTIRF) and ensemble assays to determine the molecular basis of this separation of function phenotype. We analyzed the kinetics of binding to different DNA structures including single-stranded intermediates found at sites of damage and replication. Our studies demonstrated that RPA binds to ssDNA in at least two modes characterized by different dissociation kinetics. We also showed that the aromatic residues contribute to the formation of the longer-lived state, are required for stable binding to short ssDNA regions, and are needed for RPA melting of partially duplex DNA structures. We conclude that stable binding and/or the melting of secondary DNA structures by RPA is required for DNA repair but is dispensable for DNA replication. It is likely that the binding modes are in equilibrium and reflect dynamics in the RPA-DNA complex. This suggests that dynamic binding of RPA to DNA is necessary for different cellular functions.
Michael Trakselis
Baylor University, USA
Title: Excluded strand control of hexameric helicase DNA unwinding
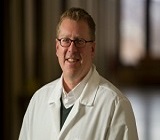
Biography:
Michael Trakselis has expertise in a number of overlapping disciplines of molecular biology, biochemical and biophysical analysis of enzymes, molecular genetics, and cell biology. His laboratory examines the molecular mechanisms and pathways of DNA helicases and polymerases involved in replication and repair. The goals are to uncover novel contacts and interactions that advance our fundamental understanding of these complex processes as well as provide novel targets for inhibition.
Abstract:
Hexameric DNA helicases are a nexus of control for both the initiation and elongation phases of DNA replication. These toroidal enzymes encircle and translocate preferentially on one strand with specific polarities dependent on their families and organismal domains. Over the past few years, there has been an increasing amount of evidence to suggest that the excluded-strand plays a significant role during unwinding by interacting with the helicase’s outer surface in a Steric Exclusion and Wrapping (SEW) mode. This excluded strand interaction appears to be dynamic and can differentially regulate the speed of DNA unwinding in diverse organisms.
We have investigated the interaction of the excluded strand with the outer surface of the bacterial, archaeal, and human hexameric helicases using a variety of biochemical, biophysical, and genetic techniques. We have not only confirmed this external interaction with the exterior surface, we can also show that this interaction regulates the DNA unwinding rate dependent on the unwinding polarity of the organism. Interaction of the lagging strand on the exterior of the archaeal homohexameric SsoMCM helicase is required for efficient and productive unwinding. We have mapped the binding orientation of the homohexameric SsoMCM onto model DNA fork substrates using site specific cleavage agents to show an intermediate in the DNA loading process.
Alternatively, interaction of the leading strand on the exterior of the bacterial DnaB helicase reduces unwinding consistent with the leading strand polymerase being intricately coupled with DnaB during normal replication conditions. In vivo genetically edited exterior mutations of dnaB have growth defects and sensitivities to DNA damage agents. Therefore, interactions with the excluded DNA strand has a previously unknown but influential role in controlling the speed of the replisome, the efficiency of DNA unwinding, and maintaining genomic integrity.
Lata Balakrishnan
Indiana University – Purdue University, USA
Title: Replication protein lysine acetylation regulates the fidelity of the human genome
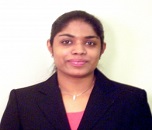
Biography:
Lata Balakrishnan is an Assistant Professor in the Department of Biology in the School of Science at Indiana University Purdue University Indianapolis. The current research in the Balakrishnan laboratory is focused on understanding the mechanistic reactions of eukaryotic lagging strand DNA synthesis, and the accompanying DNA repair processes. She trained with Dr. Robert Bambara, at the University of Rochester for her post-doctoral fellowship and received her Ph.D. under the mentorship of Dr. Barry Milavetz at University of North Dakota, wherein her dissertation work defined the epigenetic code during viral transcription.
Abstract:
The process of DNA replication needs to occur with extremely high accuracy and efficiency. Mutations incorporated into the genetic code, can cause downstream errors in the creation of proteins, which carry out functional activities within the cell. This in turn directly impacts human health, promoting oncogenesis and faster progression to cancer1. In eukaryotic nuclear DNA replication, the leading strand is synthesized continuously, but the lagging strand must be made as short Okazaki fragments that are later joined. Maturation of the lagging strand is an inherently complex process involving multiple enzymes2. We have found many proteins associated with DNA replication and/or repair to be post-translationally modified by lysine acetylation. Nick translation allows for the displacement of short flaps, followed by cleavage by FEN1 and subsequent ligation. The nuclease activity of FEN1 is diminished on lysine acetylation3. In contrast, acetylation of DNA polymerase delta and Pif1 create longer flaps in the cell, owing to an increase in strand displacement synthesis and helicase function respectively. The acetylated form of RPA binds these displaced flaps with high affinity. Stimulated nuclease function of acetylated Dna2 allows for efficient processing of the flaps for ligation4. Similarly, acetylation of many proteins in the base excision repair pathway promotes the displacement of a longer patch of damaged bases. Additionally, we found that in human cells repair efficiency was diminished in the absence of acetylation5. The displacement of longer flaps would ensure the removal of mismatched bases synthesized by the error-prone DNA polymerase alpha during the maturation phase and damaged nucleotides during the repair process. We propose that lysine acetylation of these proteins acts as a regulatory mechanism that ensures the optimal functioning of the replication/repair associated proteins in a manner that is consistent with promoting genome stability.
- Genetic Diseases
Location: Canada
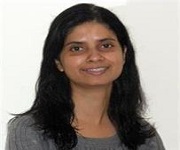
Chair
Sudha Sharma
Howard University, USA
Session Introduction
Alexandra Sobeck
University of Minnesota, USA
Title: Crosstalk between the Fanconi Anemia and ATRX/DAXX histone chaperone pathways promotes homologous recombination mechanisms in human cells
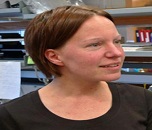
Biography:
Abstract:
The Fanconi Anemia (FA) pathway functions to repair DNA interstand crosslinks (ICLs). During ICL repair, the central FANCD2 protein promotes the recruitment of downstream factors such as BRCA2[FANCD1] and RAD51[FANCR] that are crucial for homologous recombination (HR) repair of DNA double stranded breaks (reviewed in (1,2)). Previous studies from our laboratory and others showed that FANCD2 also promotes the HR-dependent recovery of hydroxyurea (HU) stalled replication forks (3-6). Intriguingly, we recently identified a new constitutive FANCD2 interactor named ATRX Alpha Thalassemia Retardation Syndrome X-linked). ATRX, in complex with DAXX (Death Domain-Associated Protein 6), acts as a histone H3.3 chaperone that plays key roles at repetitive DNA sequences, including replication fork recovery (7-10). Here, we investigated if FANCD2 and ATRX/DAXX functionally interact to promote the HR-dependent recovery of stalled replication forks, possibly in a histone chaperone-dependent manner.
To dissect cellular roles of FANCD2 and ATRX, we generated isogenic FANCD2-, ATRX- and FANCD2/ATRX-double deficient cell lines using CRISPR/Cas9 gene targeting techniques; moreover, we used siRNA-mediated DAXX knockdown. A dual labeling DNA fiber assay was used to analyze replication fork restart after hydroxyurea (HU)-triggered replication stress. To visualize the recruitment of HR factors to stalled replication forks, we performed immunostaining experiments in the absence/presence of HU.
We found that FANCD2 and ATRX cooperate to promote replication fork restart in concert with DAXX and the MRE11 nuclease. Moreover, we discovered that ATRX supports the FANCD2-dependent recruitment of key HR factors such as CtIP, BRCA2 and RAD51 to stalled replication forks. Importantly, our results also indicate that HR-mediated replication fork restart relies on histone chaperone activities of the FANCD2-ATRX/DAXX complex. Our study suggests that FANCD2 and the ATRX/DAXX complex are involved in chromatin remodeling/histone chaperone processes that take place in the vicinity of stalled replication forks to mediate HR-dependent fork repair.
Oleg Tikhodeyev
Saint Petersburg State University, Russia
Title: The DNA theory of inheritance in the scope of protein inheritance
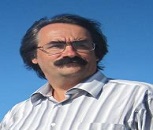
Biography:
Oleg N Tikhodeyev is the author of the original approach for resolving multiple ambiguities and contradictions in current genetic concepts. He has shown that the key source of such ambiguities and contradictions is the erroneous belief that the same genetic term (for example, mutation) is able to comprise both specific phenomenology and the underlying mechanisms (Tikhodeyev, 2015). This belief became widely accepted after 1952, when the hereditary role of DNA had been demonstrated. In modern genetic concepts, the terms describing molecular mechanisms should be clearly distinguished from those describing phenomenology because there is no strict correlation between phenomenology and molecular mechanisms (Tikhodeyev, 2016).
Abstract:
Statement of the Problem: It has long been accepted that any hereditary factor in any organism is represented by DNA sequences. This idea became fundamental in molecular genetics, and was implicitly transformed into the DNA theory of inheritance. All basic genetic terms (genotype, gene, allele, mutation, recombination, etc.) were considered as specific DNA sequences or their alterations. However, multiple examples of stable epigenetic inheritance lacking any distinctions in DNA sequences were recently discovered, and the most exciting among them is protein inheritance. Amyloid hereditary prions in fungi were considered as “protein-only” hereditary factors, which features were determined entirely by protein conformation. As a result, the principal question arises whether the DNA theory of inheritance is wrong or not.
Methodology & Theoretical Orientation: Considering different variants of the same hereditary prion as prion alleles, we examined the molecular nature of such variety.
Findings: To perpetuate stably in cell generations a certain prion allele requires two entities: specific state of the prion protein, and the corresponding DNA sequence to provide reproduction of the prion particles. We name these entities as the DNA determinant and the epigenetic determinant, respectively. Thus, a certain prion allele is a bimodular hereditary system depending on both the DNA determinant and the epigenetic determinant. Alteration of any of these two determinants may result in establishment of a novel prion allele. Moreover, similar regularities are characteristic to all other cases of epigenetic inheritance, irrespective to the underlying mechanisms.
- CRISPR Biology & Technology
Location: Canada
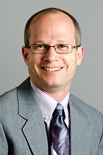
Chair
Mark Sutton
University at Buffalo, USA
Session Introduction
Rick Russell
University of Texas at Austin, USA
Title: Kinetic basis for DNA target specificity of CRISPR-Cas12a
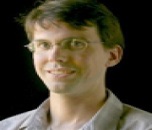
Biography:
Rick Russell has expertise in quantitative biochemical and biophysical approaches to dissect the activities of enzymes that manipulate RNA and DNA structures. Work from his lab has focused on RNA helicase proteins that function as chaperones in the complex tertiary folding processes of structured RNAs, and additional research has increased our understanding of the folding mechanisms of these RNAs. Recent work from the lab has also included a focus on DHX36, a human helicase protein that efficiently disrupts G-quadruplex structures in DNA and RNA.
Abstract:
Class II CRISPR-Cas nucleases are programmable via a guide RNA, enabling genome editing applications in nearly all organisms. However, DNA cleavage at off-target sites that resemble the target sequence is a pervasive problem that remains poorly understood mechanistically. Here, we use quantitative kinetics to dissect the reaction steps of DNA targeting by Acidaminococcus sp Cas12a (known previously as Cpf1). We show that Cas12a binds DNA tightly in two kinetically-separable steps. Protospacer-adjacent motif (PAM) recognition is followed by rate-limiting R-loop propagation, leading to inevitable DNA cleavage of both strands. Despite the functionally irreversible binding, Cas12a discriminates strongly against mismatches between the DNA target sequence and the crRNA along most of the target, implying substantial reversibility during R-loop formation and a transition state that is much later than for formation of an isolated helix in solution. Dissociation of Cas12a occurs on the time scale of hours, which together with the binding rate constant indicates an overall binding equilibrium constant of 50 fM for a fully matched DNA target. Mismatches throughout the R-loop exert variable effects on the equilibrium, ranging from 10- to 2000-fold weaker binding, with no discernable trend along the target sequence. The ability of Cas12a to discriminate against mismatches throughout the target sequence and the large energetic effects of PAM-distal mismatches defy common descriptions of a ‘seed’ sequence for Cas12a. Further, they raise the possibility of a kinetic origin, rather than a thermodynamic one, for observations of seed sequences in other CRISPR-Cas nucleases including the well-studied Cas9 nuclease. Together, our results provide a quantitative physical underpinning for the DNA cleavage patterns of Cas12a measured in vivo and observations of greater target specificity of Cas12a than Cas9.